Preparation of tube-shaped electrode materials for lithium-ion batteries is a trending topic. Tubes with hollow cylindrical bodies allow exposure of the electrodes’ interior surface and can accommodate the large volumetric expansion commonly observed when lithium ions diffuse (either via intercalation or alloying) into the electrodes. The aforementioned two characteristics improve the specific capacity (a measure of how much electric energy one electrode can hold) and lifetime of electrodes.
Recently, the Mai research group from Wuhan University of Technology, China demonstrated a straightforward method for the synthesis of tin dioxide nanotubes as high-performance anodes for lithium-ion batteries. They adopted manganese(III) oxyhydroxide (MnOOH) nanowires as the sacrificial templates and immersed them in a batch of aqueous solutions containing tin(II) cations and oxalate anions (C2O42-). Afterwards, they warmed the mixture at 60 oC under constant magnetic stirring for 4 h and collected a white precipitate consisting of tin dioxide nanotubes. These nanotubes were then washed and coated with carbon thin films to improve their electrical conductivity and structural stability before being subjected to performance evaluations.
The presence of oxalate anions was crucial for producing the nanotubes with a well-defined shape. The function of these anions was revealed through a series of experiments. Oxalate anions first reduced MnOOH to manganese(II) cations and consumed protons in the vicinity of the MnOOH surface. The consumption of local protons increased the local pH and triggered precipitation and oxidation (by dissolved oxygen) of Sn2+ to tin dioxide. The two reactions proceeded, and eventually the MnOOH nanowires disappeared but tubes of tin dioxide formed around their surfaces (Figure 1). Samples obtained without oxalate salts were irregularly shaped.
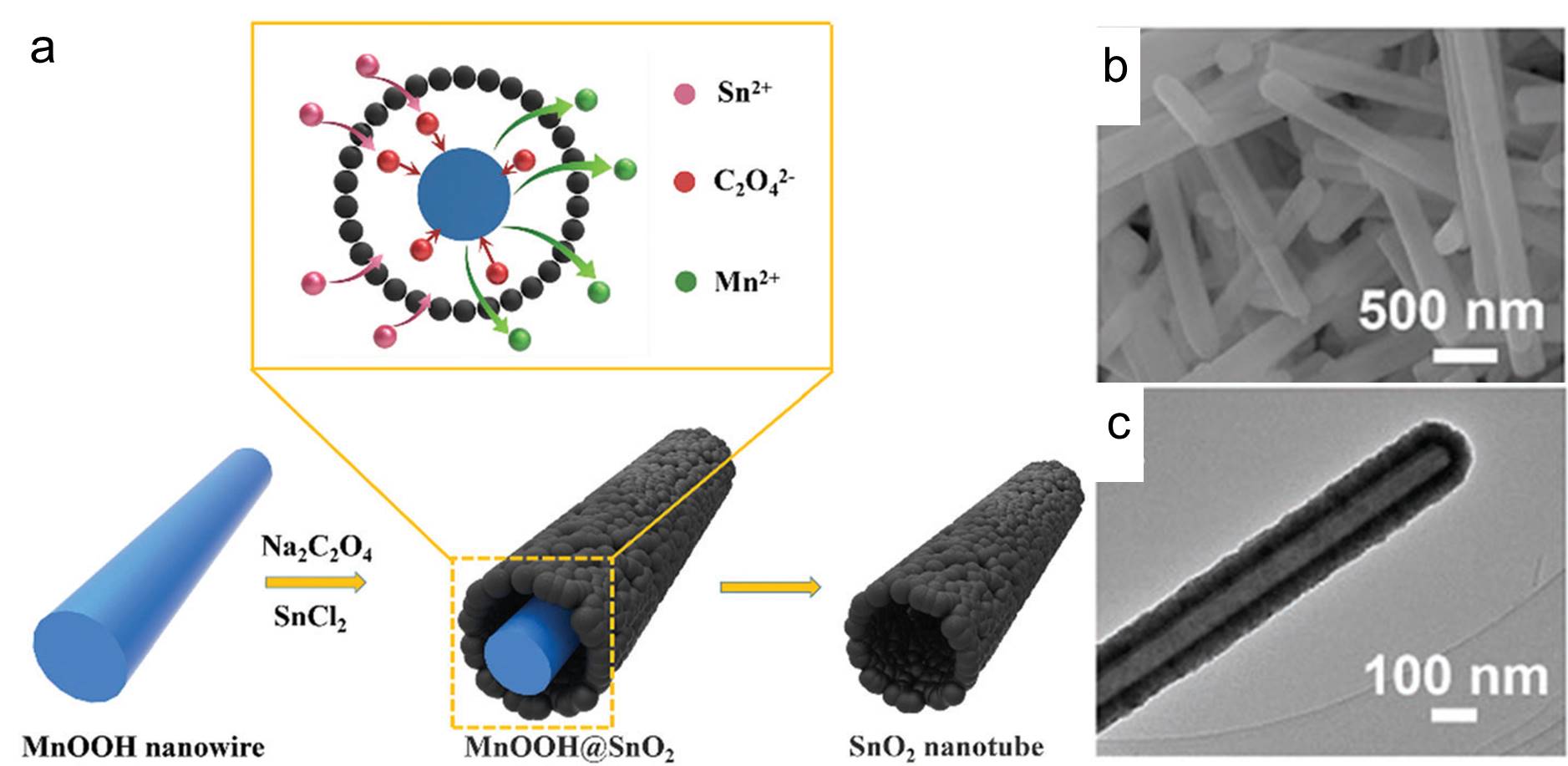
Figure 1. (a) The schematic illustration of the synthesis steps of the tin dioxide nanotubes. (b) Scanning electron microscopy and (c) transmission electron microscopy images of the as-prepared tin dioxide nanotubes.
The carbon-coated tin dioxide nanotubes showed superior stability performance to bare tin dioxide nanotubes, as shown from the slower capacity-fading rate depicted in Figure 2a. In addition, carbon coating did not significantly sacrifice nanotubes’ charge-storage performance as both electrodes with and without a coating exhibited comparable capacity at all tested current densities (Figure 2b).

Figure 2. Performance comparison between carbon-coated tin dioxide nanotubes (SnO2@C NTs) and bare tin dioxide nanotubes (SnO2 NTs): (a) long-term stability and (b) capacity achieved at different current densities and charge-discharge cycle numbers.
To find out more please read:
Oxalate-assisted Formation of Uniform Carbon-confined SnO2 Nanotubes with Enhanced Lithium Storage
Chunhua Han, Baoxuan Zhang, Kangning Zhao, Jiashen Meng, Qiu He, Pan He, Wei Yang, Qi Li and Liqiang Mai
DOI: 10.1039/c7cc05406h
About the blogger:
Tianyu Liu is a Ph.D. in chemistry graduated from University of California, Santa Cruz in United States. He is passionate about scientific communication to introduce cutting-edge researches to both the general public and the scientists with diverse research expertise. He is a web blogger for the Chem. Commun. and Chem. Sci. blog websites. More information about him can be found at http://liutianyuresearch.weebly.com/.