People who love the taste of garlic are often annoyed by its lingering smell. While there are various tips to get rid of this unpleasant odor, have you ever thought that this garlic aroma brings you chemical compounds that are potent anti-cancer reagents?
Diallyl trisulfide, one of the natural occurring components rendering the flavor of garlic, is able to release hydrogen sulfide (H2S) upon contacting with thiol compounds (i.e., organic molecules with –SH functional groups). H2S is a pungent gas that one might never forget after sniffing a rotten egg. However, this “notorious” gas, when at low concentrations, is reported to be friendly to our bodies. It relaxes vascular smooth muscle, reduces blood pressure, lowers risk associated with cancer as well as protects gastrointestinal, nervous and immune systems. All the aforementioned benefits of H2S have aroused worldwide efforts in developing H2S-releasing and bio-compatible materials that mimic the natural products for pharmaceutical applications.
Davis, Quinn and co-workers from Monash University, Australia and University of Warwick, United Kingdom, recently published a paper in Chemical Communications that reports a trisulfide-linked organic polymer capable of releasing H2S when meets –SH groups. As shown in the scheme below, the synthesized polymer is composed of three parts: a polyethylene glycol (PEG) unit on the left (in blue), a cholesterol (CHOL) group on the right (in orange), and a linker (in black) joining the two ends. PEG and CHOL are chosen mainly due to their bio-compatibility. By changing the structure of the middle linker, the authors obtained three types of polymers that behave differently when mixing with thiol compounds. The trisulfide linker (denoted as T) enables release of H2S gas and initiates polymer degradation. The disulfide linker (denoted as D) allows polymer degradation only. The amide linker (denoted as C) containing no sulfide atoms is inert to the thiol exposure.
Experiments showed that the T-linked polymers are capable of releasing H2S both in vitro and in vivo.
A fluorescent probe, which can be reduced by H2S and becomes fluorescent, is applied to detect the existence of H2S. As shown in Figure a, the trisulfide linked polymers tested in vitro exhibited the highest fluorescence when mixing with L-cysteine (a thiol compound to trigger H2S generation). For the in vivo measurements, the authors incubated HEK293 cells with the polymers and the probe. Similar as the in vitro results, the fluorescence intensity of the cells containing the T-linked polymers is the highest (Figure b). Both the in vitro and in vivo results unambiguously proved that the presence of the T-linker was responsible for generating H2S. Additionally, another set of tests using Nile Red confirmed the biodegradability of the T-linked polymers.
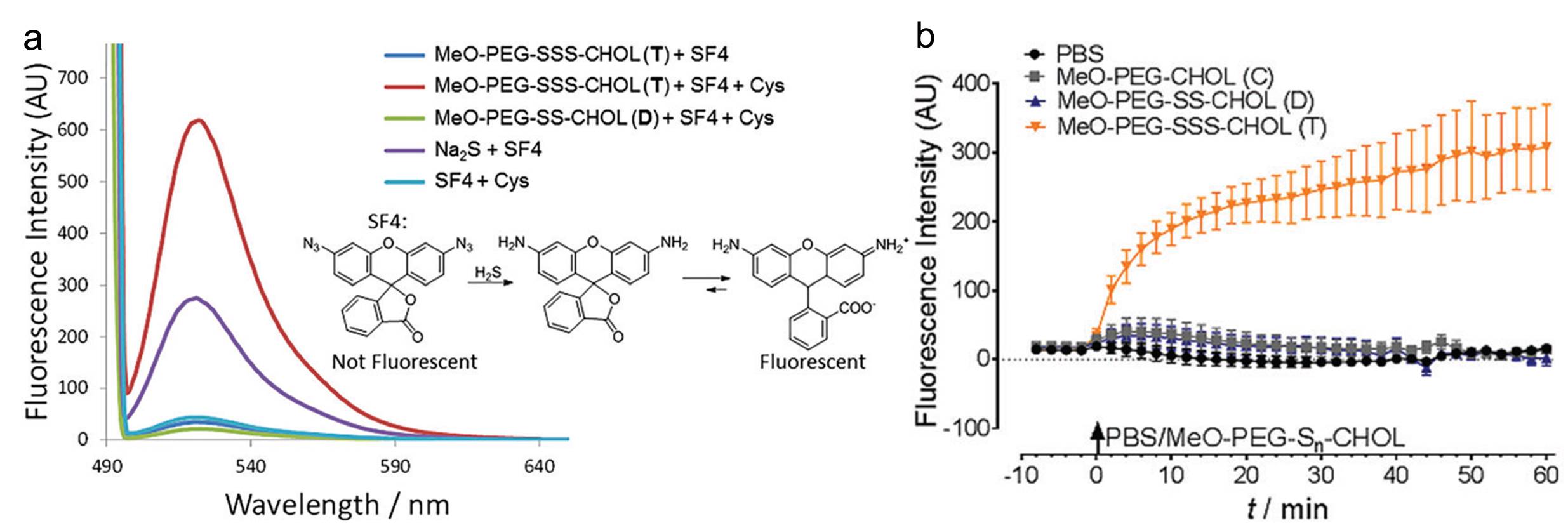
Figure. (a) Fluorescence spectra collected from different systems in vitro. The inset shows the chemical reaction between the probe (SF4) and H2S that displays fluorescence. (b) Fluorescence intensity of different polymers over time in HEK293 cells.
The developed tri-sulfide linker may allow the mimicry of endogenous biosynthesis, the initiation of discrete signaling events and the synthesis of next-generation pharmaceutical excipients.
To find out more please read:
Garlic-inspired Trisulfide Linkers for Thiol-stimulated H2S Release
Francesca Ercole, Michael R. Whittaker, Michelle L. Halls, Ben J. Boyd, Thomas P. Davis and John F. Quinn
DOI: 10.1039/c7cc03820h
About the author:
Tianyu Liu is a Ph.D. in chemistry graduated from University of California-Santa Cruz. He is passionate about scientific communication to introduce cutting-edge researches to both the general public and the scientists with diverse research expertise. He is a web writer for the Chem. Commun. and Chem. Sci. blog websites. More information about him can be found at http://liutianyuresearch.weebly.com/.