
We are delighted to highlight some of the latest cryosphere chemistry studies published in Environmental Science: Processes & Impacts.
This themed collection, Guest Edited by Rose Cory and Kerri Pratt (University of Michigan), showcases studies on chemical processes in sea ice, snow, glaciers, ice sheets and permafrost soils. This includes atmospheric chemistry (atmospheric aerosols and trace gases) biogeochemistry (chemical weathering and organic matter chemistry) as well as laboratory, field and modeling studies.

Atmospheric chemistry is understudied in the Cryosphere, cold regions of the Earth that are seasonally or continually covered with snow and ice, yet these regions represent areas of significant climate change. Snow and sea ice are sources and sinks of atmospheric trace gases and aerosols, with impacts on surface albedo, cloud formation and properties, air quality, and meltwater. The critical need to understand air-ice interactions in these cold regions is exemplified by the emerging international activity The Cryosphere and Atmospheric Chemistry (CATCH), supported by IGAC and SOLAS, which aims to facilitate atmospheric chemistry research within the international community, with a focus on natural processes specific to cold regions of the Earth. This ESPI collection includes results of recent laboratory and field studies of the interactions between the snow, ice, and overlying atmosphere, described by Kirpes et al., Ruggeri et al., Hullar et al., and Hara et al.
An outstanding CATCH question surrounds the locations, kinetics, and mechanisms of reactions on and within snow grains, as this knowledge is required to understand and simulate air-ice interactions. Hullar et al. present a laboratory study of the photodegradation of guaiacol in solution, ice, and at the air-ice interface, showing that photodegradation rate is faster within liquid-like regions in ice and especially at the air-ice interface and therefore cannot be approximated by bulk solutions. This work further demonstrates the uniqueness of reactions occurring on snow and ice surfaces in cold regions and the need for future study, both in the field and through fundamental laboratory studies.
The cryosphere contains about twice the amount of carbon found in our atmosphere, in the form of organic carbon locked away in a deep freeze in permafrost soils (perennially frozen ground). As permafrost soils warm and thaw, the organic carbon in these soils decomposes into the greenhouse gases carbon dioxide (CO2) and methane (CH4). Release of CO2 and CH4 from thawed permafrost soils will raise global temperatures beyond what our fossil-fuel-based carbon emissions would do on their own. For example, current models predict a loss of permafrost that could raise global temperatures by an additional 0.3 to 0.4 °C by 2100; a feedback called Arctic Amplification of climate change.
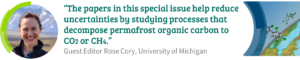
However, there is much uncertainty in these models because the processes that control the decomposition of permafrost organic carbon to CO2 and CH4 remain poorly understood. The papers in this collection help to reduce uncertainties by studying processes that decompose permafrost organic carbon to CO2 or CH4. For example, in thawed soils, microbially-mediated redox reactions convert organic carbon to CO2 or CH4. These redox reactions depend on the availability of electron donors and acceptors in soils, which in turn, vary by landscape position and hydrology (Philben et al.). Redox reactions in permafrost soils also control the availability of nutrients like phosphorous, that in turn will help regulate the potential of these soils to store or release carbon as they thaw (Herndon et al.).
As permafrost soils thaw, organic carbon in the soil dissolves and flows into the many lakes across the Arctic landscape. As evidence mounts that arctic lakes are strong sources of greenhouse gases from the cryosphere to the atmosphere, more questions emerge about the timing and drivers of these gas fluxes. The paper by Eugster et al. is the first to show that while gas fluxes vary during the ice-free season and across years, no large episodic events associated with spring ice-off or other mixing events occurred over 6 years of continuous eddy flux measurements in a deep arctic lake. However, that may change in the future, as more permafrost organic carbon flows into lakes.
Gagne et al. showed that permafrost organic carbon is rapidly converted to CO2 once exposed to sunlight. Exposure of permafrost organic carbon to sunlight in lakes is inevitable as permafrost soils thaw and export this ancient carbon into increasingly ice-free waters. And, Ward and Cory show that our concerns don’t stop with the complete oxidation to CO2. Soil organic carbon is also partially oxidized by sunlight, which in turn controls its susceptibility to complete oxidation to CO2.
Finally, the interactions between permafrost soils and receiving lakes are featured in a synthesis paper by Burpee and Saros, highlighting key knowledge gaps on the feedbacks between loss of the cryosphere on land and in water.
With permafrost loss already under way across the Arctic, we need more research in this area of cryosphere chemistry to predict the Arctic Amplification of climate change and impacts on society. A 2016 Scientific American article by John Berger summarized it best: “The faster these gases emerge from the permafrost, the less carbon human society can release and still keep global temperatures from rising far above the aspirational temperature targets set by the Paris accord.”

Comments Off on Cryosphere chemistry – Themed Collection in ESPI