Liquid-phase exfoliation (LPE) is a cheap, scalable and facile way to produce graphene nanosheets. However, what is gained in processability, is then lost in homogeneity of the resulting nanomaterial. This issue is particularly relevant in devices for which inter-nanosheet resistance plays a significant role in their intrinsic performance. For instance, piezoresistive nanosheet-based strain sensors have already been shown to be deeply influenced by network composition and morphology.
In this recent work by Caffrey et al., the influence of nanosheet thickness was investigated and correlated to the piezoresistance of printed graphene sensors. Firstly, the nanosheet suspension was prepared via LPE, different flake sizes were selected through liquid cascade centrifugation (LCC) and their thickness was then estimated via atomic force microscopy (AFM). The team produced different sensors via spray coating a network of graphene nanosheets with thicknesses between 3 and 20 nm (Fig. 1).
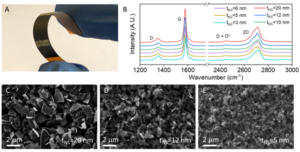
Fig 1. (A) Photograph of a printed sensor. (B) Raman spectra of sprayed graphene films inks of different size-selected nanosheets. (C)–(E) SEM images of networks composed of nanosheets with different size ranges. Reproduced from DOI: 10.1039/D4NH00224E with permission from the Royal Society of Chemistry.
The devices show a clear trend of increasing resistivity and gauge factor with increasing thickness. By using a simple model that correlates the network resistivity with nanosheet thickness, a new model that successfully correlates the gauge factor with thickness was obtained (Fig. 2).
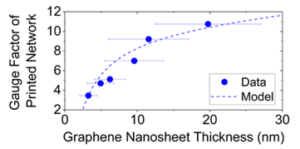
Fig 2. Plot of gauge factor as a function of nanosheet thickness showing both experimental data and the model fitting. Reproduced from DOI: 10.1039/D4NH00224E with permission from the Royal Society of Chemistry.
The authors carefully analyzed the different contributions to the gauge factor and were able to differentiate between the effect of straining of the nanosheets themselves and of the inter-nanosheet junctions. From fitting this model, they observed that, interestingly, strain has a significant influence on nanosheet resistivity, which means that applied strain not only makes the flakes in the network slide on each other, but also induces a detectable deformation on the flakes themselves. Unexpectedly, the calculated nanosheets’ gauge factor was negative. This was attributed to a decrease in nanosheet strain with applied strain, possibly due to the relaxation of built-in strain during network formation or the release of point-to-basal plane contacts.
By applying a theoretical approach to experimental data, the authors were able to interpret and quantify the piezoresistive response in disordered graphene networks produced by LPE. Overall, this study presents a step forward in actively understanding the mechanisms behind the piezoresistive behavior of printed graphene sensors.
To find out more, please read:
Quantifying the effect of nanosheet dimensions on the piezoresistive response of printed graphene nanosheet networks
Eoin Caffrey, Jose M. Munuera, Tian Carey and Jonathan N. Coleman
Nanoscale Horiz., 2024, Advance Article
About the blogger
![]()
Sara Domenici is a PhD student at Politecnico di Torino (Turin, Italy) under the supervision of Prof. Teresa Gatti. She was born in Verona (Italy) in 1998. In 2020, she obtained her Bachelor’s degree in Chemistry at the University of Padova (Italy). In 2022, she completed the Double Degree Programme between the University of Padova and the Justus-Liebig University in Giessen (Germany), where she spent 12 months, and obtained a Master’s Degree in Chemistry. Her PhD project is focused on Janus two-dimensional materials for energy conversion, but she also works on hydrogel sensors and dye-sensitized solar cells (DSSCs). |