Before the 1700’s, when dissection techniques were not yet available, the cause of mood changes were thought to be the replacement of liquids and vapors in the body. The biology of the brain has been better understood since the discovery of research and test tools. However, occupying only about 1/50 of the body mass, the brain is perhaps the most complicated organ to study. National Institute of Neurological Disorders and Stroke’s list of over 400 neurological disorders can be seen as a sound proof of this exciting- and frustrating- fact.
Biomechanical forces on neurons play a fundamental role in neuronal physiology, which, in turn, affect brain development and disorders. During the growth of neurons, the tension created by the biomechanical forces are suggested to influence the cells’ motor activities, gene expression, neurotransmitter release, together with neurite growth and network connections (Figure 1). Research on the biomechanical forces can definitely help us to understand how the brain works, but many questions related to these forces remain unanswered. Quantitative measurements of the cell activity seem to be the only possible path to find satisfying answers to those questions.
A comprehensive list of experimental techniques involving both conventional and alternative micro&nanotechnology approaches have been recently brought to the attention of scientific community by Di Carlo and his coauthors. In their recent critical review, both advantages and disadvantages of conventional tools—namely motor-driven pressure, patch membrane pressure, osmotic pressure, fluid shear stresses, and deformation of flexible elastomers—, microtechnology tools—including atomic force microscopy, micropatterning, and some other potential techniques—, and nanotechnology tools—such as ferromagnetic and piezoelectric nanoparticles— are discussed.
The literature reports provided in the paper suggest that micro and nanotechnology tools offer better spatiotemporal resolution and throughput when compared to conventional techniques. The cellular functions and the possible technologies for the characterization of those functions are further described (Figure 2). For instance, behind-the-scene biological mechanisms for recovery in traumatic brain injuries can be determined by applying the biomechanical forces at the right place and right time to ultimately mitigate the injuries.
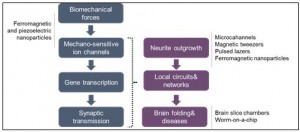
Figure 2. The influence of biomechanical forces on the neuron functions and available technologies for their investigation.
This article, published on 26 April 2016, is included in the Lab on a Chip Recent HOT Articles themed collection.
To download the full article for free* click the link below:
Micro- and nano-technologies to probe the mechano-biology of the brain
Andy Tay, Felix E. Schweizer, and Dino Di Carlo
Lab Chip, 2016,16, 1962-1977
DOI: 10.1039/C6LC00349D, Critical Review
—————-
Burcu Gumuscu is a PhD researcher in BIOS Lab on a Chip Group at University of Twente in The Netherlands. Her research interests include development of microfluidic devices for second generation sequencing, organ-on-chip development, and desalination of water on the micron-scale.
—————-
*Access is free until 15/08/2016 through a registered RSC account.