These HOT articles have been recommended by our referees and are free to access for 4 weeks*
Multiplexed single molecule immunoassays
David M. Rissin, Cheuk W. Kan, Linan Song, Andrew J. Rivnak, Matthew W. Fishburn, Qichao Shao, Tomasz Piech, Evan P. Ferrell, Raymond E. Meyer, Todd G. Campbell, David R. Fournier and David C. Duffy
DOI: 10.1039/C3LC50416F

Rapid fabrication of pressure-driven open-channel microfluidic devices in omniphobic RF paper
Ana C. Glavan, Ramses V. Martinez, E. Jane Maxwell, Anand Bala Subramaniam, Rui M. D. Nunes, Siowling Soh and George M. Whitesides
DOI: 10.1039/C3LC50371B
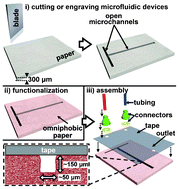
A simple three-dimensional-focusing, continuous-flow mixer for the study of fast protein dynamics
Kelly S. Burke, Dzmitry Parul, Michael J. Reddish and R. Brian Dyer
DOI: 10.1039/C3LC50497B
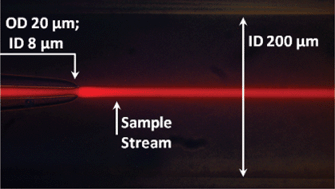
Assessment of pathogenic bacteria using periodic actuation
Sorin David, Cristina Polonschii, Mihaela Gheorghiu, Dumitru Bratu, Alin Dobre and Eugen Gheorghiu
DOI: 10.1039/C3LC50411E
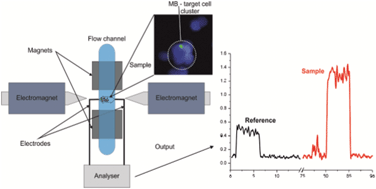
Microfluidic heart on a chip for higher throughput pharmacological studies
Ashutosh Agarwal, Josue Adrian Goss, Alexander Cho, Megan Laura McCain and Kevin Kit Parker
DOI: 10.1039/C3LC50350J
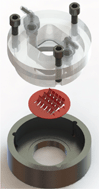
Low-cost fabrication of centimetre-scale periodic arrays of single plasmid DNA molecules
Brett Kirkland, Zhibin Wang, Peipei Zhang, Shin-ichiro Takebayashi, Steven Lenhert, David M. Gilbert and Jingjiao Guan
DOI: 10.1039/C3LC50562F
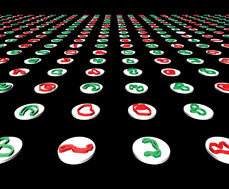
A novel microfluidic technology for the preparation of gas-in-oil-in-water emulsions
Lu Yang, Kai Wang, Sy Mak, Yankai Li and Guangsheng Luo
DOI: 10.1039/C3LC50652E
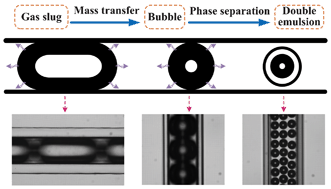
A microfluidic approach for protein structure determination at room temperature via on-chip anomalous diffraction
Sarah L. Perry, Sudipto Guha, Ashtamurthy S. Pawate, Amrit Bhaskarla, Vinayak Agarwal, Satish K. Nair and Paul J. A. Kenis
DOI: 10.1039/C3LC50276G
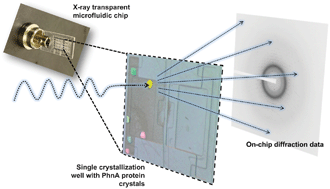
Steam-on-a-chip for oil recovery: the role of alkaline additives in steam assisted gravity drainage
Thomas W. de Haas, Hossein Fadaei, Uriel Guerrero and David Sinton
DOI: 10.1039/C3LC50612F
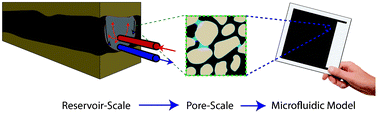
Out of the cleanroom, self-assembled magnetic artificial cilia
Ye Wang, Yang Gao, Hans Wyss, Patrick Anderson and Jaap den Toonder
DOI: 10.1039/C3LC50458A
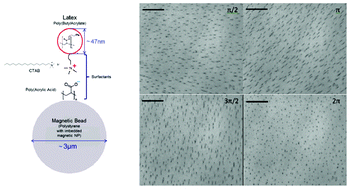
Flow switching in microfluidic networks using passive features and frequency tuning
Rachel R. Collino, Neil Reilly-Shapiro, Bryant Foresman, Kerui Xu, Marcel Utz, James P. Landers and Matthew R. Begley
DOI: 10.1039/C3LC50481F
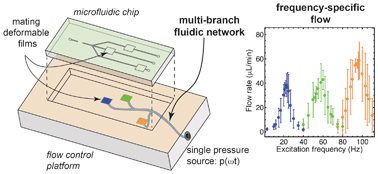
Single vesicle biochips for ultra-miniaturized nanoscale fluidics and single molecule bioscience
Andreas L. Christensen, Christina Lohr, Sune M. Christensen and Dimitrios Stamou
DOI: 10.1039/C3LC50492A
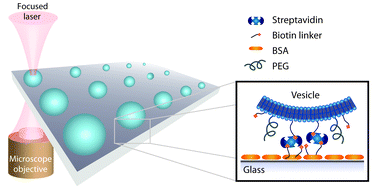
Pinched-flow hydrodynamic stretching of single-cells
Jaideep S. Dudani, Daniel R. Gossett, Henry T. K. Tse and Dino Di Carlo
DOI: 10.1039/C3LC50649E
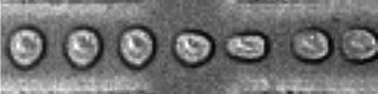
An acoustofluidic micromixer based on oscillating sidewall sharp-edges
Po-Hsun Huang, Yuliang Xie, Daniel Ahmed, Joseph Rufo, Nitesh Nama, Yuchao Chen, Chung Yu Chan and Tony Jun Huang
DOI: 10.1039/C3LC50568E
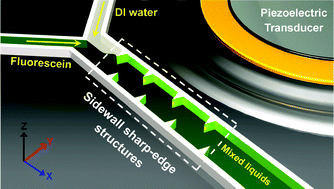
Thermal migration of molecular lipid films as a contactless fabrication strategy for lipid nanotube networks
Irep Gözen, Mehrnaz Shaali, Alar Ainla, Bahanur Örtmen, Inga Põldsalu, Kiryl Kustanovich, Gavin D. M. Jeffries, Zoran Konkoli, Paul Dommersnes and Aldo Jesorka
DOI: 10.1039/C3LC50391G
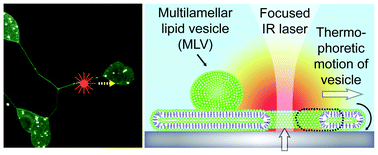
On-chip microbial culture for the specific detection of very low levels of bacteria
Sihem Bouguelia, Yoann Roupioz, Sami Slimani, Laure Mondani, Maria G. Casabona, Claire Durmort, Thierry Vernet, Roberto Calemczuk and Thierry Livache
DOI: 10.1039/C3LC50473E
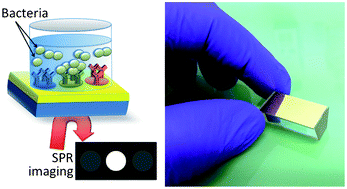
Gas/liquid sensing via chemotaxis of Euglena cells confined in an isolated micro-aquarium
Kazunari Ozasa, Jeesoo Lee, Simon Song, Masahiko Hara and Mizuo Maeda
DOI: 10.1039/C3LC50696G
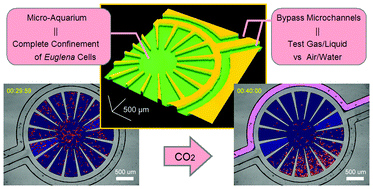
Smart-phone based computational microscopy using multi-frame contact imaging on a fiber-optic array
Isa Navruz, Ahmet F. Coskun, Justin Wong, Saqib Mohammad, Derek Tseng, Richie Nagi, Stephen Phillips and Aydogan Ozcan
DOI: 10.1039/C3LC50589H
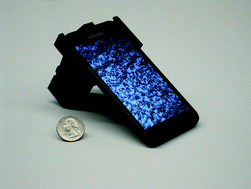
Protein–DNA force assay in a microfluidic format
Marcus Otten, Philip Wolf and Hermann E. Gaub
DOI: 10.1039/C3LC50830G
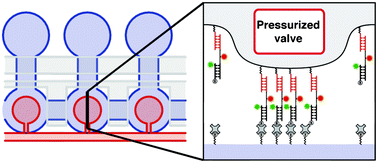
Ultrasensitive microfluidic solid-phase ELISA using an actuatable microwell-patterned PDMS chip
Tanyu Wang, Mohan Zhang, Dakota D. Dreher and Yong Zeng
DOI: 10.1039/C3LC50783A
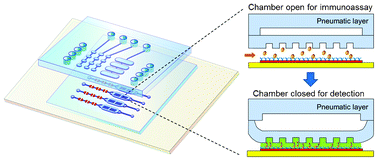
Detection of real-time dynamics of drug–target interactions by ultralong nanowalls
Andreas Menzel, Raphael J. Gübeli, Firat Güder, Wilfried Weber and Margit Zacharias
DOI: 10.1039/C3LC50694K
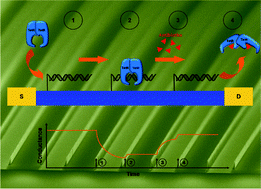
Capillarics: pre-programmed, self-powered microfluidic circuits built from capillary elements
Roozbeh Safavieh and David Juncker
DOI: 10.1039/C3LC50691F
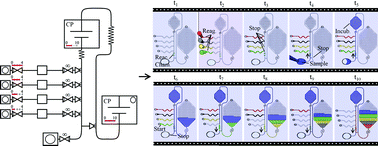
A portable explosive detector based on fluorescence quenching of pyrene deposited on coloured wax-printed μPADs
Regina Verena Taudte, Alison Beavis, Linzi Wilson-Wilde, Claude Roux, Philip Doble and Lucas Blanes
DOI: 10.1039/C3LC50609F
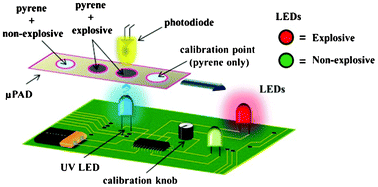
Electrokinetic tweezing: three-dimensional manipulation of microparticles by real-time imaging and flow control
Zachary Cummins, Roland Probst and Benjamin Shapiro
DOI: 10.1039/C3LC50674F
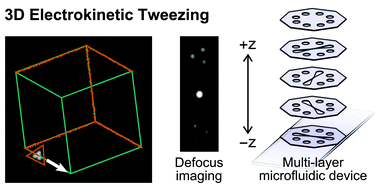
Albumin testing in urine using a smart-phone
Ahmet F. Coskun, Richie Nagi, Kayvon Sadeghi, Stephen Phillips and Aydogan Ozcan
DOI: 10.1039/C3LC50785H
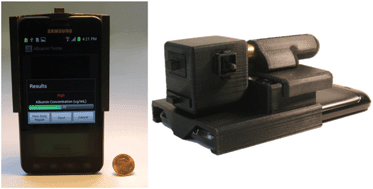
*Free access to individuals is provided through an RSC Publishing personal account. It’s quick, simple and more importantly – free – to register!