The synthesis and control of the performance of chiral superstructures are intriguing because they bring us closer to the magical concept of chirality, exemplified by the dichotomy between our hands. In the long run, scientists aim to control the chirality of matter, particularly molecules. The enduring challenge is to overcome the mismatch between the molecular work function and magnetic quadrupole transitions and/or the wavelength of light. “Due to this size mismatch, the molecule sees uniform electric and magnetic fields, just as we see the Earth as locally flat,” said Prof. Adam E. Cohen in one of his pioneering reviews on chirality (Nano Today (2009) 4, 269—279). The design of chiral superstructures allows us to sculpt the three-dimensional shape of the electromagnetic field at the size scale of an individual molecule. The most rapidly developing strategy to prepare these nanostructures involves using diverse nanoscale templates, such as origami techniques or lithography. However, the preparation of such nanostructures is very challenging, which slows down the field’s development. Another significant limitation is the inability to dynamically control the handedness and spectral characteristics.
Recent work by Chaolumen Wu and Yadong Yin suggests a magnetic assembly strategy to overcome these limitations. They prepared Ag@Fe3O4 nanoparticles through a relatively simple procedure and further assembled them by introducing a chiral magnetic field from a cubic permanent magnet. This magnet was placed beside the Ag@Fe3O4 suspension and was controlled by two parameters: rotation angle and distance to the suspension (shorter separation distance results in a stronger field strength). The formed assemblies are chains of nanoparticles oriented in a chiral structure. The main advantage of the suggested strategy is the very smoothly controlled dynamicity of the system: spectral position, handedness, and intensity of the chiral signal can be controlled by the magnetic angle and distance relative to the sample. Previously reported approaches required time-consuming simulations and production of plasmonic substrates with those varied chiral characteristics. The authors could fix the chiral superstructures in polymer films with precise control of the handedness, position of optical absorption, and degree of plasmonic coupling. The dynamic optical rotation enables the authors to demonstrate distinguishable color switching. Without the magnet, no color is observed; however, variation of magnet positions gives a wide palette from purple to pink, and from yellow to blue. Therefore, the authors mainly envision the application of this tunability for color-changing optical devices used in anti-counterfeiting and stress sensors.
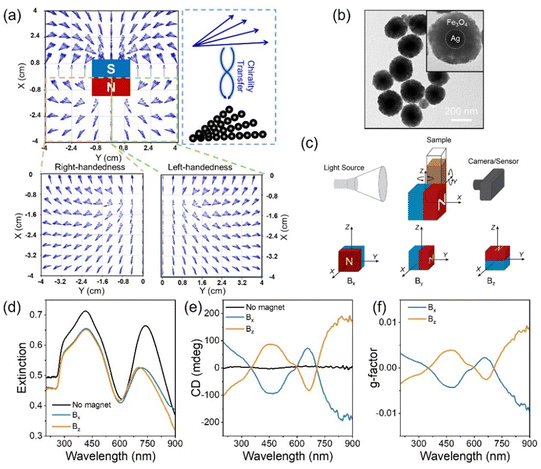
Figure 1: (a) Simulation of the chiral field distribution from a cubic permanent magnet and schematic illustration of the chirality transfer from a chiral magnetic field to magnetic nanoparticle assembly. (b) TEM image of Ag@Fe3O4 hybrid nanoparticles. (c) Schematic illustration of the setup for measuring the extinction and CD spectrum of particle dispersion under a chiral magnetic field. (d)–(f) Extinction (d), CD (e), and the corresponding g-factor (f) spectra of the Ag@Fe3O4 nanoparticle dispersion without a magnetic field or under a field along the X- and Z-axes. Reproduced from DOI: 10.1039/D3MH01597A with permission from the Royal Society of Chemistry
However, the author of this blog highlights alternative applications. Controlling chiroptical properties is a direct way to enable enantioselective sensing and catalysis by transferring chirality to small molecules. While the application of a chiral quadrupole magnetic field induces the assembly of Ag@Fe3O4, simultaneous irradiation of these structures with a wavelength corresponding to the maximum absorption, due to the presence of Ag, could enhance interactions with small molecules. Photocatalytic reactions performed under a magnetic field, which couple magnetic and light fields, are a novel concept. Although magnetic field-enhanced photocatalysis is relatively new and has mostly been applied to dye degradation, controlling chiral photochemistry with a magnetic field would significantly advance interactions with chiral molecules. In this scenario, applied magnetic fields to Ag@Fe3O4 could serve a dual role of (i) chirality induction and (ii) plasmonic carrier generation and prolongation of the lifetime of excited plasmons.
Research on chirality remains niche due to the complex preparation techniques of chiral superstructures. The published research opens new possibilities for more scientific groups to work in this direction, thanks to the simplicity of using cubic permanent magnets. However, measurement techniques, such as optical rotation density measurement setups, may still pose challenges for the wider community. Increased availability of these techniques should spur more investigations into various applications of chiral nanostructures, including color displays, anti-counterfeiting measures, and chiral sensing and catalysis.
To find out more, please read:
Magnetic assembly of plasmonic chiral superstructures with dynamic chiroptical responses
Chaolumen Wu, Qingsong Fan, Zhiwei Li, Zuyang Yea and Yadong Yin
Mater. Horiz., 2024,11, 680-687, DOI: 10.1039/D3MH01597A
About the blogger
![]()
Dr Olga Guselnikova is a member of the Materials Horizons Community Board. She recently joined the Center for Electrochemistry and Surface Technology (Austria) to work on functional materials as a group leader. Dr. Guselnikova received her PhD degree in chemistry from the University of Chemistry and Technology Prague (Czech Republic) and Tomsk Polytechnic University (Russia) in 2019. Her research interests are related to surface chemistry for functional materials. This means that she is applying her background in organic chemistry to materials science: plasmonic and polymer surfaces are hybridized with organic molecules to create high-performance elements and devices.
|