Materials Horizons are delighted to welcome Professor Paulette Clancy as the newest member of the Advisory Board.
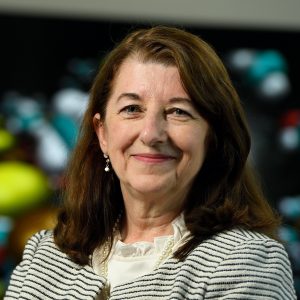
Paulette Clancy is the Edward J. Schaefer Professor of chemical and biomolecular engineering. More recently, she became the director of research for the AI-X Foundry. She is the Associate Director of the Johns Hopkins Center for Integrated Structure-Mechanical Modeling and Simulation (CISMMS), and a fellow of the Hopkins Extreme Materials Institute (HEMI) and AIChE. She spent over 30 years teaching at Cornell before moving to Johns Hopkins in 2018 to become the inaugural department Head of ChemBE.
Clancy leads one of the top groups in the country studying atomic- and molecular-scale modeling of semiconductor materials, ranging from traditional silicon-based compounds to all-organic materials. Her group’s research comprises four main areas: advanced organic materials (covalent organic frameworks, antibacterial oligomers, organic electronics); algorithm development (force field development, machine learning, and Bayesian optimization); electronic materials (particularly III-IV semiconducting materials; and nucleation and crystal growth (hybrid organic/inorganic perovskites and quantum dot nanocrystals). Her lab focuses on studies of advanced materials processing and nucleation, including understanding the links between processing, structure, and function.
Her group is at the forefront of developing new Bayesian optimization methods to encode expert knowledge and intuition, creating optimal conditions for making energy-efficient solar cells, close-to-perfect quantum dots, and discovering polymorphs of electronic materials for shape memory applications.
She is a fierce long-term advocate for the increased representation of women and those from groups under-represented in engineering and the physical sciences. She was the founding chair of a faculty group, “Women in Science and Engineering” for Cornell University. Among her awards for that advocacy is the American Institute of Chemical Engineers (AIChE) National Women’s Initiatives Mentoring Award. She is a member of the mentoring team for Project Elevate, a DEI initiative between Hopkins in partnership with NYU and CMU.
Check out some of Paulette’s recent RSC publications:
A comprehensive picture of roughness evolution in organic crystalline growth: the role of molecular aspect ratio
Jordan T. Dull, Xiangyu Chen, Holly M. Johnson, Maria Clara Otani, Frank Schreiber, Paulette Clancy and Barry P. Rand
Mater. Horiz., 2022,9, 2752-2761, DOI: 10.1039/D2MH00854H
A new metric to control nucleation and grain size distribution in hybrid organic–inorganic perovskites by tuning the dielectric constant of the antisolvent
Blaire A. Sorenson, Lucy U. Yoon, Eric Holmgren, Joshua J. Choi and Paulette Clancy
J. Mater. Chem. A, 2021,9, 3668-3676, DOI: 10.1039/D0TA12364A
A multiscale approach to uncover the self-assembly of ligand-covered palladium nanocubes
Xiangyu Chen, Thi Vo and Paulette Clancy
Soft Matter, 2023, Advance Article, DOI: 10.1039/D3SM01140B
Read our interview with Paulette below:
What does it mean to you to join the Advisory Board of Materials Horizons?
I feel honored to join the Advisory Board because this is such an exciting and relatively new journal. It brands itself as being “transformative” and that’s how I have found the research that it publishes. I am glad to be part of the team to keep up the wonderful momentum that it has.
What is the current biggest challenge you face in your field?
I work in the area of machine-learning guided materials discovery. The biggest challenge I currently face is to sift through the burgeoning number of new methods in this hot area and learn which ones are truly exciting and ground-breaking.
Why do you feel that researchers should choose to publish their work in Materials Horizons?
MH has it all: Thoughtful and helpful reviewers, short time to triage and review, and careful selection of strong papers.
Can you tell us about one of your latest Materials Horizons publications?
I actually have some of my most exciting new Bayesian optimization algorithm development under review with MH right now, so fingers crossed for that one. My last paper involved a joint computational (us)-experimental (Princeton) study of the thin-film growth of molecules that could be used for electronic devices. To function well in that regard, you need to create films that are as smooth as possible. Our paper looked at a few candidates for new electronic materials and showed that you need to take a holistic view of the growth process rather than relying just on the traditional step-edge energy barriers to arbitrate between rough (unwanted) and smooth growth (desirable). We were thrilled to be recognized with an “Editor’s Choice” designation and the cover. |
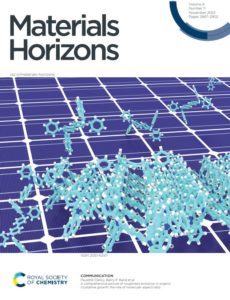
Paulette’s last Materials Horizons article was featured on the issue front cover |
Please join us in welcoming Paulette to the Materials Horizons Advisory Board!