We are delighted to introduce our latest Lab on a Chip Emerging Investigator, Ashleigh Theberge!
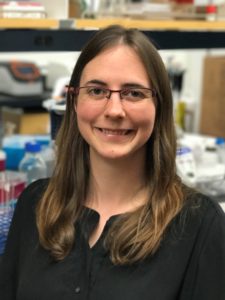
Ashleigh Theberge is an Assistant Professor of Chemistry at the University of Washington and Adjunct Assistant Professor of Urology at the University of Washington School of Medicine. She received her BA in Chemistry at Williams College and her PhD in Chemistry at the University of Cambridge, UK with Wilhelm Huck. During her graduate work, she was a Visiting Scientist with Andrew Griffiths at the Université de Strasbourg, France. Her graduate research focused on droplet-based microfluidics for chemical synthesis and analysis. She completed her postdoctoral fellowship in Biomedical Engineering and Urology with David Beebe, William Ricke, and Wade Bushman at the University of Wisconsin-Madison. In 2014, she was awarded an NIH K Career Development Award from the NIDDK in Urology. She joined the faculty at the University of Washington in 2016. Her group develops new microscale culture and analysis methods to study cell-cell, cell-extracellular matrix, and host-microbe interactions, with a focus on inflammation in urologic diseases and asthma.
Read Ashleigh’s Emerging Investigator series paper “Upgrading well plates using open microfluidic patterning” and find out more about her in the interview below:
Your recent Emerging Investigator Series paper focuses on upgrading well plates using microfluidic patterning. How has your research evolved from your first article to this most recent article?
My research has changed dramatically since my first article, which focused on chemical synthesis in droplet-based microfluidics. The common thread in both articles is manipulation of fluids on the microscale, but almost everything else is different! While my graduate work focused on chemical applications of microfluidics in conventional closed microfluidic channels, I now work on methods for studying cell signaling in biological systems using primarily open microfluidic channels. For example in this most recent article, the “walls” of the “channel” are comprised of the floor of the well plate, a plastic insert, and two free air-liquid interfaces.
What aspect of your work are you most excited about at the moment?
I am excited about our group’s fundamental work advancing fluid manipulation in open microfluidics using capillary flow. On the applications side, I am excited to be exploring signaling in ever-more complex cellular systems, including “multikingdom” systems where we study the chemical signals exchanged between host and microbe. I am also happy to work with a fantastic group of students who are taking our group in new directions both biologically and technologically.
In your opinion, what is the biggest advantage of the presented patterning device over the current systems?
Our method enables biologists to easily “upgrade” any existing assay developed in conventional well plates to a co- or multiculture assay. Well plates are the most common cultureware used for cell-based assays, and our device is a simple plastic insert that fits within the well and enables the user to pipette hydrogels (such as collagen, Matrigel, or synthetic gels) to make biocompatible partitions that enable segregated cell culture and maintain soluble factor signaling through the gel wall. Our device enables biologists to use a familiar off-the-shelf platform (well plates) and culture cells on surfaces that have been optimized by decades of industry experience with tissue culture treated surfaces. Since our device uses “open microfluidics,” it does not require bonding of multiple layers and can be fabricated in a single step via injection molding to scale up production.
What do you find most challenging about your research?
A challenge is the interdisciplinary of our work; but this is also what I love about it. Much of our work involves new methods to isolate and study chemical signals from complex cultures – falling under the category of analytical chemistry or bioengineering. But we also focus heavily on disease mechanisms, delving deeply into biology, and our work in the fundamentals of open microfluidics is at the interface of physics and chemistry. Our work also touches on applied aspects of microscale device fabrication, traditionally falling under mechanical engineering, such as our recent paper in press at Lab on a Chip entitled “Fundamentals of rapid injection molding for microfluidic cell-based assays.”
In which upcoming conferences or events may our readers meet you?
I regularly attend microTAS and the Gordon Research Conference on Microfluidics. I also plan to attend the IEEE EMBS Micro and Nanoengineering in Medicine Conference and biological conferences related to our group’s focus areas (microbiome, urologic research, etc.).
How do you spend your spare time?
I try to spend spare time outdoors and enjoy sailing, open water swimming, and climbing. I also like to travel – both to explore different cultures and also wilderness such as the Yukon Territory, Patagonia, and national forest land nearby in Washington.
Which profession would you choose if you were not a scientist?
I would enjoy having a leadership role in a company or organization. I like brainstorming ideas to develop a vision for large projects and working in teams toward a common goal – be it a product, a discovery, or a process. I decided to be a scientist because the scientific method provides an opportunity to rigorously explore questions, but there are many complex problems outside of science that require exciting new strategies and the cohesive effort of many minds to solve.
Can you share one piece of career-related advice or wisdom with other early career scientists?
One strategy that has been helpful for me is to be persistent in looking for opportunities to support projects that I care about. As an undergraduate researcher, I designed my own project that required augmenting our department’s gas chromatograph. I was able to secure the funds by talking with different offices on campus (dean’s office initiatives, student project grants, etc.); I was turned down many times but even those experiences helped me to refine my explanation of the project to have a more compelling conversation with the next person I approached. As an undergraduate summer intern working for Merck, my department did not have funds to send me to a conference to present my work, so I asked the human resources department to fund my trip as a way to promote the intern program as well as present my science; they agreed. In graduate school, I set up an international collaboration in order to develop the expertise required to pursue a multidisciplinary project that I had designed. So for early career scientists, I think it is key to remember that there are many opportunities (even if they are not always obvious at first), and facing road blocks or being turned down several times doesn’t preclude a successful outcome.