To celebrate the growth and development of the RSC Applied Polymers community and to highlight the remarkable authors who continue to contribute their high quality work to the journal we would like to share the opinions and insights of these authors through this introductory blog post. Once dubbed #RSCAppliedfirst50, our blog posts aim to give a voice to the authors behind the research and hope that their insights might shed light upon growing challenges and progress in polymer science and its applications.
In this edition, we hear from Professor Seth C. Rasmussen in a short interview about their study ‘Sidechain engineering in poly(2,3-alkylthieno[3,4-b]pyrazine)s via GRIM polymerization: Solubility, film formation, and device performance’
An introduction to ‘Sidechain engineering in poly(2,3-alkylthieno[3,4-b]pyrazine)s via GRIM polymerization: Solubility, film formation, and device performance’ by Professor Seth C. Rasmussen
How would you summarise the direction of your research presented in your paper?
This paper was focused on improving the solution processability of poly(thieno[3,4-b]pyrazine)s, which are a class of low bandgap (Eg < 1.5 eV) polymers with bandgaps down to 0.7 eV. Prior to this work, the best example of these materials was poly(2,3-dihexylthieno[3,4-b]pyrazine) produced via GRIM polymerization, which is a type of polymerization via Ni-catalyzed Kumada coupling. The work here produced high quality analogues that utilized branched 2-ethylhexyl sidechains instead of the previous simple straight-chain hexyl groups. As hoped, this significantly increased the processability and film formation properties of the polymer, along with significantly improved device performance, although it did not result in greater molecular weights as expected. As a result, this led to a new understanding of the polymerization method used to generate these materials and a newly proposed catalyst poisoning mechanism that accounts for all of the results produced to date.
What had inspired you to research into conjugated polymers for photodetectors?
Due to the very low bandgaps of poly(thieno[3,4-b]pyrazine)s (i.e., Eg = 0.9-1.1 eV for processable species), these are ill suited for conventional photovoltaic devices (solar cells). This is due to the Shockley-Queisser limit, which predicts that as the polymer bandgap diminishes below about 1.34 eV, more and more absorbed energy is lost to heat, and the device efficiency will decrease as a consequence. However, our earliest devices exhibited photo response down to 1200-1300 nm, which is something that only a few conjugated polymers can do. As such, this naturally led to applications in NIR photodetectors. Unlike conventional photovoltaic devices, NIR photodetectors are less dependent on power conversion efficiency (PCE) and their effectiveness is determined more by what wavelengths can be detected and the corresponding detectivity (D*(l)) at those wavelengths. As such, this is a natural fit for these materials, as this most recent paper aptly demonstrates. NIR photodetectors are critical to optical communications, remote control, night time surveillance, and medical monitoring, and the ability to make such devices from organic plastics offers possibilities not possible with conventional inorganic materials.
What aspect of your work on conjugated organic materials are you most excited about at the moment?
Much of our focus over the last several years has involved our discovery that the conjugated building block theino[3,4-b]pyrazine (TP) is not a simple acceptor unit as has been commonly believed. While it does have a low lying LUMO and does act as a strong acceptor, it also has a high lying HOMO and provides a donor strength roughly equivalent to 3,4-ethylenedioxythiophene (EDOT). As such, we have proposed the term ambipolar unit to such species that simultaneously act as both donors and acceptors. Not only has this new insight into its electronic properties finally allowed a deeper understanding of the trends observed in donor-acceptor (D-A) frameworks utilizing TP as the acceptor, but has also led to the understanding that even TP homopolymers such as those reported in the current paper are also technically D-A frameworks, with the thiophene acting as the donor and the pyrazine the acceptor. Furthermore, this has also opened up completely new design paradigms for low bandgap polymers, as demonstrated by our research work utilizing TP as the donor and pairing it with traditional acceptors to generate polymers with bandgaps of ca. 1 eV and deep LUMO levels (ca. -3.8 to -3.9 eV) (10.1039/d5tc00519a). The more we can do to advance our fundamental understanding of the various species utilized to produce conjugated polymers, the more effectively the field as a whole can move forward to further develop more advanced materials.
In your opinion, what are the most important questions to be asked/answered in your field of research?
In our view, one of the most important issues in the field of conjugated materials is the growing synthetic complexity of current state of the art materials. The field as a whole has made great strides in the production of high-performance materials capable of ever-increasing device performance, yet this has been coupled with significant increases in the structural and synthetic complexity of the materials applied. Many of these materials can be composed of three or more different monomeric units, with the total synthesis requiring multiple sequential steps to control the desired order of monomer connectivity, as well as any potential regiochemistry involved, resulting in as much as 10+ synthetic steps for their production. As such, the important question to be asked is can high-performance materials be produced with more reasonable levels of synthetic complexity? This is critical as current trends have resulted in materials that are far to costly to make for commercial viability. While we personally feel that this is possible, it will require a shift in viewpoint within the field such that device performance is not the sole focus of research efforts, with greater emphasis also placed on the practical synthetic accessibility and scale of the materials involved. As a representative example, the poly(thieno[3,4-b]pyrazine) reported in the current paper has a calculated synthetic complexity index of 35.8, while the bulk of the other top-performing materials capable of NIR detection beyond 1000 nm have significantly higher values of 75.1-92.5. While our material is not the best performer, it is still among the best and demonstrates that high-performing materials are possible with significantly lower synthetic complexity and thus reduced production costs.
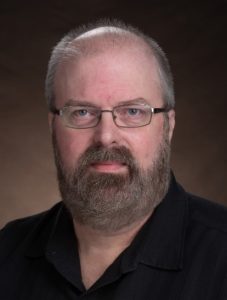
Seth C Rasmussen
Seth C. Rasmussen is Professor of Chemistry at North Dakota State University (NDSU). Educated at Washington State University (B.S. 1990) and Clemson University (Ph.D. 1994 with John Petersen), he then worked as a postdoc with James Hutchison at the University of Oregon. He became an instructor at Oregon in 1997, before moving to NDSU in 1999. Professor Rasmussen also spent the spring of 2018 as a Fulbright Senior Scholar and visiting professor at the Centre for Organic Electronics of the University of Newcastle, Australia. At NDSU, he has developed extensive research on the synthesis and design of conjugated materials, particularly those with low (Eg < 1.5 eV) and reduced (Eg = 1.5-2.0 eV) bandgaps. As a noted chemist-historian, he also studies the history of materials and is considered the leading authority on the history of conjugated polymers. He was named a Fellow of the American Chemical Society in 2021, a Fellow of the Royal Society of Chemistry in 2022, and his work in history was recognized with the 2025 Joseph B. Lambert HIST Award for Outstanding Achievement in the History of Chemistry.
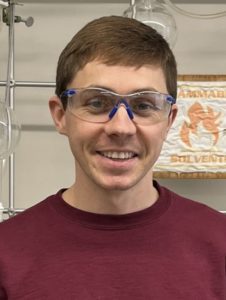
Spencer J. Gilman
Spencer J. Gilman earned his B.S. in chemistry from St. Cloud State University in 2018 and his Ph.D. from North Dakota State University in 2024 under the guidance of Seth Rasmussen, where his dissertation focused on the synthesis and characterization of conjugated materials for near-infrared emitters and photodetectors. He is currently a postdoctoral researcher at the Georgia Institute of Technology under the mentorship of John Reynolds, specializing in conjugated materials with a focus on electrochromics and postpolymerization modification.
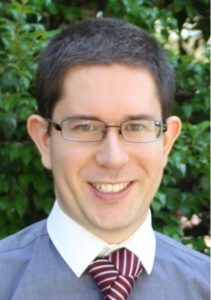
Nicolas Nicolaidis
Nicolas Nicolaidis completed his PhD thesis focussed on the optical properties of organic photovoltaics and upscaling their production in 2015 with the Centre for Organic Electronics, supervised by Prof. Paul Dastoor. On completion, he continued to work in the group on industrial projects and continuing to scale up the technology. He typically provides support and expertise to equipment to characterise solar materials such as the UV-vis spectrometer, fluorimeter, AM1.5 solar simulator, and an external quantum efficiency measurement system. In upscaling the organic photovoltaic devices, he has been significantly involved in mechanical prototyping, electronic design, and software design to successfully deliver large projects with international impact and significance.
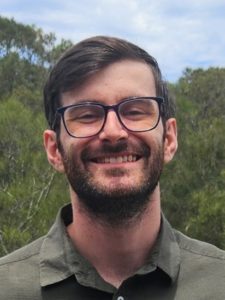
Tomas Marsh
Tomas Marsh is a Ph.D. student at the University of Newcastle, Australia, working with the Centre for Organic Electronics. He also obtained his Bachelor of Science with Honours there in 2021. His research focuses on optimisation of organic photovoltaics and applications of ultrafast spectroscopy.
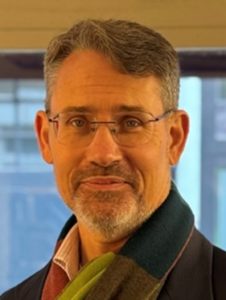
Paul Dastoor
Paul Dastoor is Professor of Physics at the University of Newcastle in Australia. He received his B.A. degree in Natural Sciences and his PhD in Surface Physics from the University of Cambridge. He is Director of the Centre for Organic Electronics, which he established in 2007. He has been Visiting Research Fellow at Fitzwilliam College, Cambridge, UK, at the Daresbury Laboratory, Cheshire, UK at Nanyang Technological University and Leverhulme Visiting Professor at the University of Cambridge. He is currently the Royal Society Wolfson Visiting Fellow at the University of Cambridge and Visiting Fellow at Fitzwilliam College and Clare Hall College, Cambridge. His research interests encompass the growth and properties of thin films, neutral atom microscopy and organic electronic devices based on semi-conducting polymers. These exciting materials offer the tantalising prospect of paints that generate electricity directly from sunlight and sensors that can be printed as flexible arrays.
Sidechain engineering in poly(2,3-alkylthieno[3,4-b]pyrazine)s via GRIM polymerization: Solubility, film formation, and device performance
RSC Appl. Polym., 2025, Advance Article
![]() |
RSC Applied Polymers is a leading international journal for the application of polymers, including experimental and computational studies on both natural and synthetic systems. In this journal, you can discover cross-disciplinary scientific research that leverages polymeric materials in a range of applications. This includes high impact advances made possible with polymers across materials, biology, energy applications and beyond.
|